In September 2018, emeritus professor of genome sciences and biology Benjamin “Ben” Hall cut the ribbon on the University of Washington’s new Life Sciences Building.
This was a gesture that did not go un-rehearsed.
“They had delivered a pair of giant scissors to his house two weeks before the dedication because Ben insisted he have time to practice. He wanted to be prepared for everything,” remembers H.D. “Toby” Bradshaw, professor and chair of UW’s Department of Biology.
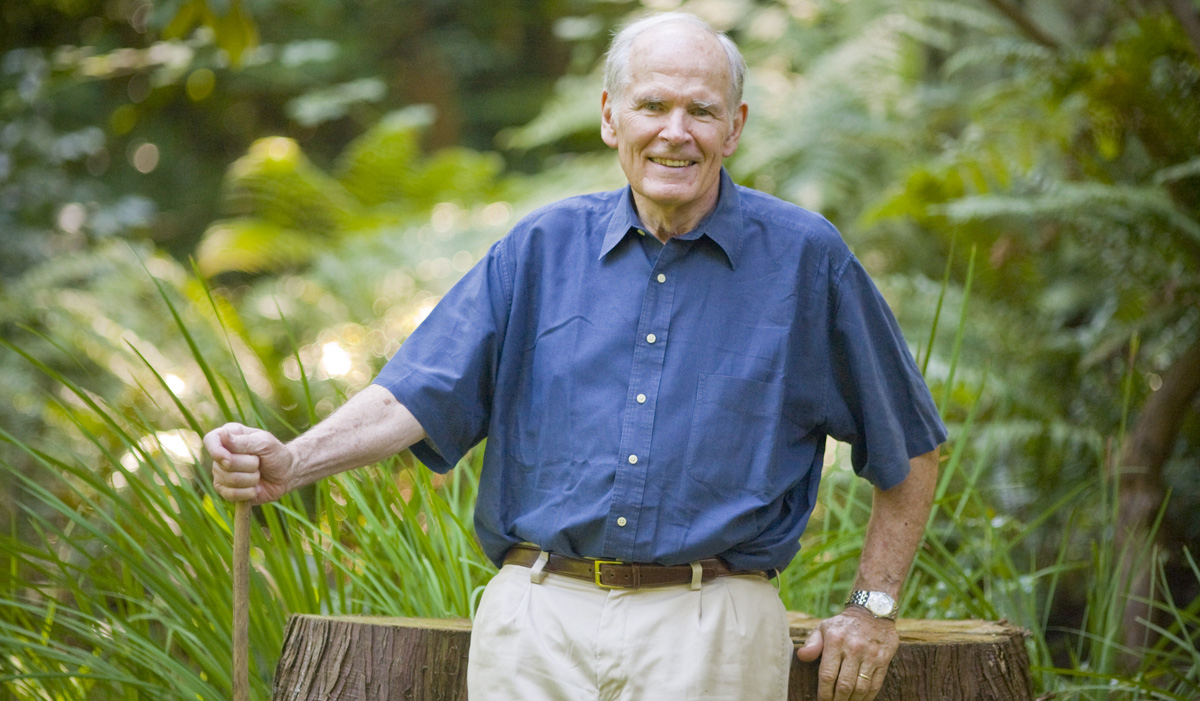
A big world out there
Hall was no stranger to making things impeccable. Yet while meticulous, he also found fulfillment in exploration and testing boundaries. His colleagues cite him as being a scientist who always saw the big picture, and who was ahead of his time.
But he was also a throwback. A romantic: the 19th century version of one, who viewed the natural world as limitless. Romanticism embraced the visionary, the transcendental, the creative.
“The word ‘amazing’ isn't a word you hear every day from a research scientist. But Ben thought the natural world was amazing, and he wanted to make his mark on trying to understand the amazing things. Once he set his mind on something, he would plunge ahead,” says Maynard Olson, Ph.D., Professor Emeritus of Medicine and Genome Sciences at UW, who worked in the Hall lab as a postdoctoral researcher in the 1970s.
But although romantics favored emotion over reason, Hall did favor an empirical approach toward nature, and the good that investigating it could bring.
Basic instincts
Basic science is simply that: practicing science for science’s sake. It’s the most fundamental study of the world around us. Basic science aims to satisfy our own curiosity about how anything works. It has no specific application to medicine or technology, and is purposefully curiosity-driven. The value of basic science lies in pursuing a scientific question, understanding that we might not initially perceive the value of that pursuit, or the outcomes that may arise from it.
“If you look at the major scientific breakthroughs, they weren’t someone saying, ‘We need to answer this particular question.’ Breakthroughs came by saying, ‘There’s a system, and we don’t know how it works, so let’s go and figure it out,’” says Jeffrey Bennetzen, Ph.D., Norman and Doris Giles Professor and the Georgia Research Alliance Eminent Scholar at the University of Georgia. Bennetzen worked with Hall from 1974-79, while completing his Ph.D.
This type of thinking was evident in Hall’s work during the birth of the field of molecular biology. After James Watson and Francis Crick correctly described the “double helix” structure of DNA in 1953, a multi-decade revolution quickly followed to understand how genetic material was stored, replicated, and used.
DNA sits in the control center of a cell—the nucleus. It never leaves. But ribonucleic acid (RNA) is a single-stranded copy of DNA; it does leave the nucleus, performing the work to make new cells and create proteins that are essential for survival. The process of making RNA copies of DNA is called transcription: A type of RNA, called messenger RNA, carries the information about how to make proteins.
Hall discovered he could put human genetic sequences, in the form of DNA, into baker’s yeast. The yeast would transcribe DNA into messenger RNA, and then translate messenger RNA into human protein. (This is considered a form of “recombinant DNA,” genetically engineered DNA that usually joins DNA from more than one species.)
“[This process] had substantial value in agricultural and biomedical applications,” Bradshaw adds.
Why yeast?

There are certain types of yeasts, including Saccharomyces cerevisiae (also known as S. cerevisiae) that are commonly used in biological laboratory settings. Yeast cells, like human cells, are eukaryotic: They have a nucleus. Although yeast doesn’t have a complex developmental process like humans do—in which cell development may lead to specialized tissues and organs—yeast and human cells do reproduce similarly.
This was important knowledge when, in the late 1970s, scientists were working to create a vaccine for Hepatitis B. The disease is transmitted sexually and by intravenous (IV) drug use, as well as from mother to child. Attempts that used small amounts of the virus to create immunity were dangerous, so researchers wanted to develop a non-infectious vaccine.
Every virus has a genetic code, with certain proteins that allow it to infect a host. The outer membrane of a virus usually contains a “coat protein”: This protects the virus’ genetic material and makes it easier for an infection to infiltrate the body’s cells. Ideally, the body’s immune system can recognize a coat protein, and fight the virus before it invades.
Before yeast was used in molecular biology, bacteria were used instead. But bacteria are prokaryote, which means they are single-celled organisms without a nucleus. For example, the bacteria E. coli is prokaryotic.
Researchers had tried to use E.coli to replicate the coat protein for Hepatitis B and create a vaccine, but it didn’t work: The bacteria grew too slowly and degraded the protein.
But then researchers discovered they could make the coat protein for Hepatitis B in yeast. Once a person is injected with these yeast-made Hepatitis B coat proteins, the person’s immune system will make antibodies, which will neutralize the virus and protect the person from infection by Hepatitis B.
“If you ever get a Hepatitis B shot, they ask if you’re allergic to yeast. This is why,” explains Bradshaw.
Patent process
There were actually two patent families around this work, says Beth Etscheid, Ph.D., director of grant programs and director of licensing at Seattle-based Washington Research Foundation (WRF).
For the first, Hall, his colleague Gustav Ammerer, and colleagues at the biotechnology company Genentech were inventors on the U.S. patent Expression of Polypeptides in Yeast (applied for in 1981 and issued in 1997). This claimed and described a method of creating genetically engineered proteins in yeast. The patent was jointly assigned to WRF and Genentech, and licensed to dozens of pharmaceutical and biotechnology companies. The technology enabled these companies to develop some of the world’s most important vaccines, such as the vaccine for the human papillomavirus (HPV), as well as therapeutic and diagnostic proteins, including insulin.
The second, Synthesis and Assembly of Hepatitis B Virus Surface Antigen, an invention by Hall, Ammerer, and colleagues Bill Rutter and Pablo Valenzuela at UCSF that resulted in a patent jointly assigned to the University of California and WRF, described how to use the yeast expression method to make the active ingredient of the Hepatitis B virus (HBV) vaccine. This patent application was filed in 1981 and issued in 2003. The vaccine made by this process is now routinely given to newborn babies in many countries, including the U.S., Europe, and China, and is credited with greatly decreasing Hepatitis B infections and the rate of liver cancer, over half of which is caused by chronic Hepatitis B infection.
The overall result was that this generated about $1 billion in royalties over the lifetime of the patents. But not only did it bring that kind of revenue and support new discoveries, it also saved millions of lives.
This all took place during a time when research scientists didn’t have a handy process that took discoveries from lab to clinic, says Bradshaw. “Ben plowed that path. Patenting intellectual property [like this] is modeled on the work he did.”
Throughout this drawn-out patenting process, which involved court cases in Europe as well as extensive U.S. review, vaccines were still being made, because companies such as Glaxo and Merck had purchased licenses for the process while the patents were pending.
“When the first patent was issued in April 1997, that was a really important moment,” says Etscheid, “It took off like a rocket.”
Although patents and licensing brought in significant monetary, scientific, and cultural change, Hall wanted to ensure his work was still accessible to everyone. He made his reagents (both yeast and DNA molecules to use in lab experiments) available at cost for nonprofit researchers. He’d send yeast strains to scientists for only the cost of postage and the technician’s time to assemble the materials.
“The overall result was that this generated about $1 billion in royalties over the lifetime of the patents. But not only did it bring that kind of revenue and support new discoveries, it also saved millions of lives. For a research scientist to see that impact in their lifetime is extremely, extremely rare,” says Bradshaw.
A new interest blooms
Hall’s more recent research centered on how rhododendron plants evolved. That work was of significant interest to him, because it posed a set of classic evolutionary questions about bio-geography: Why are species distributed the way they are?
His work involved trying to reconstruct the genetics and origins of rhododendrons, globally. Hall and his wife Margaret traveled all over the world—including to remote areas of China and Southeast Asia—to study different species of this plant.
“Studying rhododendrons was the capstone to his career,” says Olson.

Rhododendrons are surprisingly diverse. They flourish in the temperate Pacific Northwest climate, where they present as substantial-sized bushes. But rhododendrons have hundreds of species; they’re found all over the world, from mountainous regions to the tropics.
Yet tropical rhododendrons look very different: They are epiphytes, growing on other plants, but not parasitic. They’re smaller than rhododendrons in temperate climates. And their flower morphology—the way they look—appears different in the tropics. How they attract pollinators varies, too.
For example, rhododendrons in the Pacific Northwest are pollinated by bees. In the tropics, they appear to be pollinated by hummingbirds, based on the color and how the flowers are shaped: long and narrow to suit a bird’s beak. They are more reddish than pink. Pollinators, as well as the plants’ genes, may both be responsible for these differences.
It was a nice way of doing outreach as a scientist: It took us outside of the university and into the public.
Hall was instrumental to the rhododendron community, including many visits to Federal Way’s Rhododendron Species Foundation & Botanical Garden (RSBG). The RSBG has one of the largest collections of rhododendrons in the world, featuring more than 700 of the more than 1,000 species found throughout North America, Europe, Asia, and Australia.
“He had a whole community he interacted with at the rhododendron garden. He served on their board and went on field trips with them to collect rhododendron samples in the wild. It was a strong community there,” says Valerie Soza, a research scientist and engineer in the Hall lab. “He would drive us down to the garden and we would stay and have lunch with them—all the volunteers and staff—after collecting our research samples. It was a nice way of doing outreach as a scientist: It took us outside of the university and into the public.”
She adds, “Now, we go there on our own.”
Generous and inspiring
Hall and Margaret were generous philanthropists, says Bradshaw. And they used their influence to ensure the majority of the money went back to basic research for the UW’s Department of Biology—the same kind of work Hall had done to make life-saving discoveries in the first place.
He would get excited—actually would get a twinkle in his eye—when we talked about how he could help the department recruit top faculty and students.
One of their philanthropic efforts at UW was to donate $6.7 million toward a vision to transform biology at the university by creating a new model of research and education that is nimble, entrepreneurial, question-focused, and team-oriented. Ben and Margaret realized this vision required two critical pieces: people and space.
“Ben was not just an investor, but a catalyst and a partner,” says UW’s Associate Dean for Advancement Tracy Ostrem. “He would get excited—actually would get a twinkle in his eye—when we talked about how he could help the department recruit top faculty and students.”
Hall’s legacy goes beyond the biology department; the revenue that the patents generated for the university has brought in approximately $500 million that’s fueled further innovation across campus. Revenue from his patents have also funded projects throughout the Puget Sound region.
According to the Washington Research Foundation, Hall’s work also helped position Seattle as a leading city in the biotechnology space.
“He was a strong proponent of the most basic research guided by the curiosity of really, really smart people. He sponsored graduate students, endowed a chair that holds his name [the Benjamin Hall Endowed Chair in Basic Life Sciences], and supported research and development of young faculty all across the university,” says Bradshaw. “He took pleasure in his own accomplishments, but it was not all important to him that he be feted.”
Hall also set the tone for giving among his colleagues. A third of the biology department’s retired faculty have created endowments, or have donated to follow the lead that Hall created, with the goal of helping the next generation of scientists.
John Stiller, Ph.D., a former graduate student of Hall’s and now an associate professor in biology at East Carolina University in Greenville, North Carolina, says, “Ben was interested in everything. He was open to new ideas and approaches, and was willing to take chances to support and promote work. Ben gave me the opportunity to explore and develop the things that I was excited about as a scientist. That was invaluable. It was both an honor and a pleasure to work with him.”
Hall’s generosity extended across the world as well. “I was at a botanical conference in China and met a student in a master’s degree program, who later contacted me when she got a scholarship to work in a U.S. lab,” says Soza. “When I approached Ben about it, he supported hosting her here. He was interested in providing opportunities for people of different backgrounds and having a culturally diverse lab.”
Roaming thoughts
Hall was known for his openness to new ideas and questioning existing ones, even if they challenged his beliefs. His background in chemistry brought him a strong understanding of the “molecular” part of molecular biology: He could look at various recombinations and understand how the various molecules would be effective.
The first scientific papers about recombinant DNA were published in the mid-1970s, and Hall was involved right away, although geneticists were initially skeptical. But Hall understood it was the future: He spent lots of time on the phone with molecular biology centers where recombinant DNA was being used, such as at Harvard and Stanford, learning about their scientific protocols. Ben’s understanding of chemistry allowed him to see how recombinant DNA would be key to understand genes.
“Ben brought great ideas into biology. People like that who are cross-disciplinary come up with interesting collaborations, which are likely to lead to invention,” says Etscheid.
In theory, major agencies that fund scientific research have the goal of taking chances with an idea, even though it may fail. But researchers feel much of that has fallen through; in order to get funding, some lament, you practically need the results of the study before you even do it. But many feel the opportunity that Hall gave scientists, to explore something—even if a complete red herring—because of the potential “what if?” was a gift.
“I can’t imagine a more fulfilling career than the kind basic scientists have. You would have to have been a medieval artist to have as much intellectual freedom as a university scientist. The way we try to repay that is by answering questions about how the world works, and communicating it to future scientists. Ben was one of the best examples of how to make the most of an opportunity like that,” says Bradshaw.
--
Carin Moonin is a freelance writer in Portland, Oregon.
More Stories
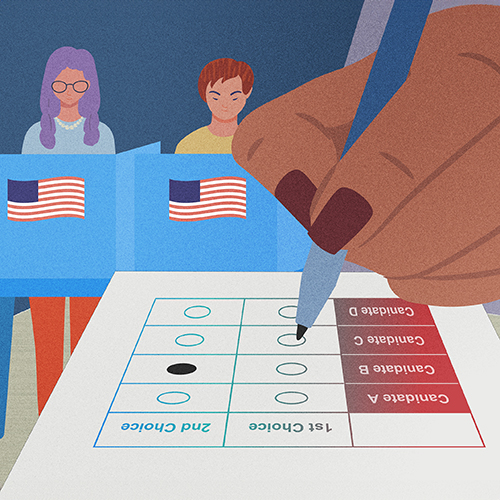
Democracy by the Numbers
Mathematics and Democracy, an undergraduate mathematics course, explores the role of math in many aspects of democracy, from elections to proportional representation.
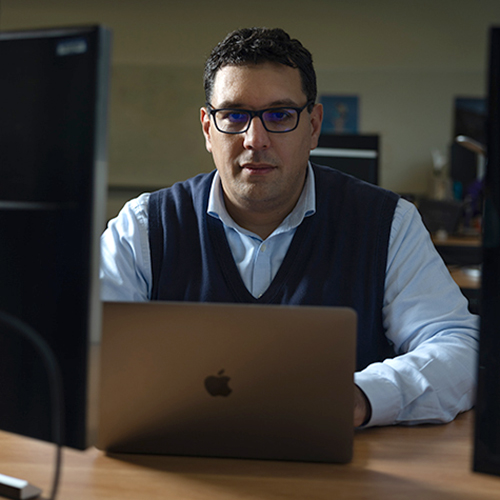
A Statistician Weighs in on AI
Statistics professor Zaid Harchaoui, working at the intersection of statistics and computing, explores what AI models do well, where they fall short, and why.
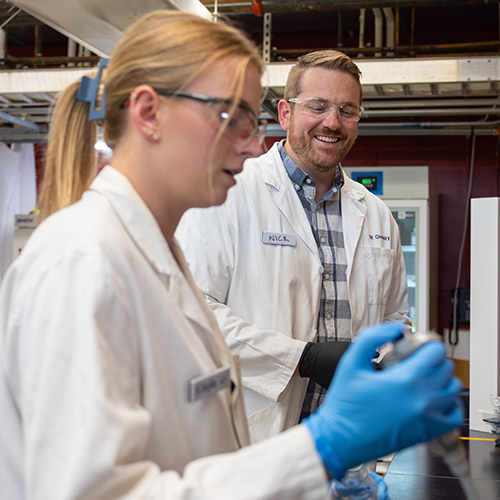
The Mystery of Sugar — in Cellular Processes
Nick Riley's chemistry research aims to understand cellular processes involving sugars, which could one day lead to advances in treating a range of diseases.